Leslie Schoop *15 is using the logic of chemistry to build quantum materials with revolutionary properties
Watch the Venture Forward video
The experts told Leslie Schoop *15 from the start that it couldn’t be done — her idea of using an unlikely chemistry lab technique to create futuristic “two-dimensional” materials.
Two Russian physicists at England’s University of Manchester won a Nobel Prize in 2010 for doing it a different way. They used a roll of sticky tape to peel flakes off a chunk of graphite crystal, then applied tape repeatedly to the flakes to remove increasingly thin nanolayers, ultimately creating a flat wonder material called graphene. Despite being essentially two dimensions — actually a single layer of carbon atoms around 200,000 times thinner than human hair — graphene was stronger than steel. By restricting its electrons to a flat layer, it made them move in unpredicted ways. Graphene could conduct electricity far more efficiently than copper.
The low-tech “Scotch-tape method” became a standard way to obtain the growing variety of 2D crystals that are a new frontier in quantum materials science, inspiring dreams of flexible electronics printed on fabrics and skin, super-efficient electricity grids, levitating trains (due to their magnetic force) and speed-limit-shattering quantum computers. But Schoop felt that ripping tape wasn’t the kind of production process that could easily scale up to create substantial quantities of material. She thought it could be done better with liquid.
“Applying Scotch tape to make a lot of materials is not really feasible,” she said. “Liquid exfoliation was used in other fields, but no one ever thought about a careful chemical recipe using it to produce quantum materials. We said we’re going to use liquid-based chemical methods to delaminate these materials, and they’re going to be awesome electronics.”
Not everyone agreed. Skeptics believed a chemical exfoliation process, which uses a solvent such as acid to break layers of a solid crystal apart, would introduce too many defects for crystals to function in electronics. Her grant proposals were repeatedly rejected, sometimes with comments suggesting the liquid idea could never work.
“Major funding agencies told me this is not possible; the process is going to be too dirty,” Schoop said.
But Princeton University’s Department of Chemistry was ready to make an audacious bet on Schoop’s vision. She had earned her Ph.D. in chemistry from Princeton in 2015 before returning to her native Germany on a fellowship. In 2017, she joined the chemistry faculty. A grant from the Princeton Catalysis Initiative got her research rolling. “Princeton likes high-risk ideas if you sound like you know how to tackle them,” Schoop said.
Schoop staffed a lab, and her team began years of experimentation and discovery — and sometimes frustration — as they tried to produce clean 2D sheets of material using chemicals. They refined their methods and materials, learned from failures and near misses. “There were review papers being published all the way up to 2022 saying it’s impossible, that damage is unavoidable in this method,” Schoop said. “And I got a job in 2017 saying I would do it!”
Possibilities at Princeton
Growing up in Aachen, Germany, Schoop interrogated the world around her.
“I liked to play witch with a cauldron and make potions,” she said. She wanted to decipher the ingredients on shampoo bottles and in her food, to understand the elements of everyday life by appreciating their molecular composition. (She once told the magazine Chemical and Engineering News that her favorite element is gold “because it is pretty and has high spin-orbit coupling.”)
After earning her undergraduate degree in chemistry in Germany, she resisted recruitment efforts by German chemical companies, suspecting corporate work might be too restrictive for her, more focused on production than discovery. Her grandfather, a medical doctor, encouraged her to pursue her passions, which led her to the United States and graduate studies at Princeton.
Her mother taught her to think about possibilities rather than impossibilities. “My mom once told me, completely unrelated to science, that there’s always a solution,” Schoop said. “If you get stuck somewhere, you miss your flight or whatever, there’s always a solution to the problem. You just need to find it.”
As a Princeton graduate student, she studied in the lab of Robert Cava, the Russell Wellman Moore Professor of Chemistry. Much of the Cava lab’s research involved building new kinds of superconductors and quantum materials, in which particles at atomic scale don’t seem to operate by the rule book of classical physics. Historically, many of these advanced materials, like graphene, have been discovered by happenstance. There wasn’t a systematic way to forecast what compounds might be good candidates to synthesize in the lab as possible superconductors. The conundrum was obvious to Schoop. “How do we find them if we can’t predict them?” she asked.
She learned to follow what chemistry researchers sometimes call “chemical intuition” — the concept that, amid the infinite ways scientists can combine elements in the search for revolutionary new materials, knowing how atoms assemble together can help isolate the most promising ideas. Her doctoral dissertation at Princeton proposed a systematic method for discovery, comparing known superconductors and finding their common characteristics. It set the trajectory for her future work.
Schoop began to win accolades, including one that returned her to Germany. In 2015, she accepted a Minerva Fast Track fellowship, which aims to increase the number of women in scientific leadership roles, at the Max Planck Institute for Solid State Research in Stuttgart. When she opted to return to Princeton, to chemistry teaching and research, the Max Planck Institute offered her a deal: they’d call her back in six years and make her a director. They were true to their word, but Schoop chose to stay in New Jersey.
“Princeton is very hard to leave,” she said. “It is a unique place that enables us to do the best in research. There’s internal funding so we can try out ambitious projects that others don’t want to risk. Our students are absolutely fantastic, and Princeton attracts extremely good postdocs. We have resources like the equipment at the Imaging and Analysis Center. Collaborations on campus are fruitful — we have really strong chemistry and physics departments. All these factors enable a lot of freedom so we can do our best work.”
In 2019, Schoop won the Beckman Young Investigator Award as one of “the most promising young faculty members in the early stages of academic careers in the chemical and life sciences.” In 2020, she added a Packard Fellowship, designed to encourage “blue-sky thinking” to foster new discoveries.
In addition to her lab, Schoop directs the Princeton Center for Complex Materials, a center funded by the National Science Foundation, where Princeton researchers from six departments in science and engineering collaborate. In both venues, her role is as a sort of lead detective, guiding teams hunting for quantum materials that may have extraordinary properties. Those include 2D topological insulators, whose ability to conduct electricity without heat loss could make them an energy-efficient substitute for silicon in computers, helping counter the exploding electricity demands of artificial intelligence and other data processing. (The U.S. Department of Energy forecasts data centers will consume as much as 12% of total U.S. electricity by 2028.)
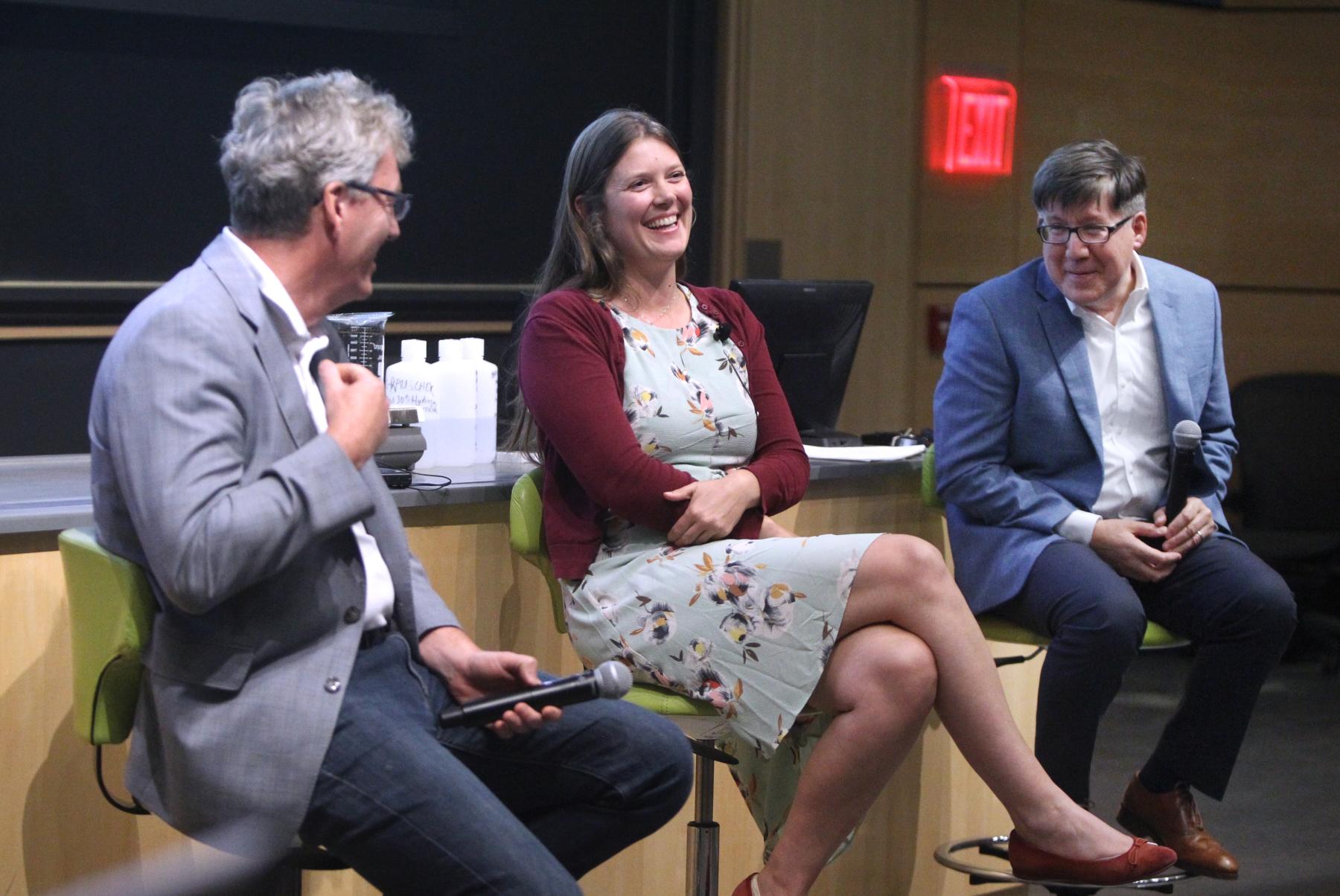
The alchemy of divining new quantum materials often involves a mind-meld between chemistry and physics, so Schoop has assembled research teams that are diverse, not just internationally but academically.
“Leslie is always open to new ideas, and that’s why she hires people from different backgrounds, so you get insights from different areas. In materials science, this exchange of ideas is very important and sometimes is missing,” said Ratnadwip Singha, a physicist who was in Schoop’s lab during the liquid exfoliation research and now runs his own condensed matter physics lab at the Indian Institute of Technology in Guwahati, India. “Most of all, she’ll never discourage you to try anything new.”
In this nascent field, where materials with unforeseen behaviors are rewriting textbooks, being open to new approaches and welcoming unpredicted results is essential, and it helps lead to breakthroughs.
What’s most interesting is what shouldn’t be there
Schoop plans her research and classes from her office in a top corner of the Frick Chemistry Laboratory. The space is austere and knickknack-free. Her awards aren’t on the walls, but there’s a small card on a coffee table displaying the periodic table of the elements, whose atomic numbers are the foundation of her investigations. “I know most of it but have to check sometimes,” she confessed.
Throughout history, materials discoveries and creations have changed civilization. Bronze, iron, steel, glass, silicon — each derives its world-changing characteristics from its unique arrangement of atoms. That is the realm of chemistry. Physicists theorizing materials on chalkboards often rely on chemists to unlock the step-by-step recipes for finding or synthesizing them. One of Schoop’s missions has been to “chemistry-fy” that search — identifying likely combinations of elements based on a knowledge of chemical reactions, synthesizing new materials in the lab and then seeing what promising electrical or magnetic properties they might have.
“Humanity knows about 50,000 different crystalline inorganic compounds that we would be interested in, but we know the properties of just a fraction of those,” she said. “If you picture it as a pyramid, there are 50,000 at the bottom and very few at the tip that actually make it into applications. Our goal is moving materials up this pyramid efficiently, not by just randomly studying materials, but formulating guidelines based on chemical logic. Billion-dollar industries are built on predictable chemical reactions. Pharma companies, chemical companies — they work on the idea that we are actually quite good in predicting where a reaction might go.”
Even backed by chemistry expertise and intuition, the discovery process is iterative and painstaking. Schoop has found it useful to pass along to her research teams the mindset that her mother gave her: there’s always a solution. You just have to find it. Sometimes finding answers means rethinking an approach you have invested a lot of time in. That’s what happened with her lab’s pursuit of liquid exfoliation and superconducting ink.
Schoop was convinced it could be done. It was just a matter of technique. Peeling thin sheets off bulk materials using liquid wasn’t radical; technicians had widely used the process to create materials for batteries and catalysts. Defects actually can help make things more catalytic, she said, so “there was never an incentive to make better sheets.”
Schoop’s team worked on methods for years, but the results they were getting in the lab were disappointing. “We exfoliated a lot of things, and the materials were poor quality,” she said. “We could still write papers about it, because they might be interesting for other applications, but it wasn’t what I really wanted to do.”
“Most of my first four years seemed to be negative results,” recalled Xiaoyu Song *22, who was Schoop’s first graduate student in 2017. “At the beginning I was almost doubting whether 2D sheets are a real thing or not. It’s nanomaterial. You cannot see it directly with your eyes.”
Finally, in 2022, the breakthrough came in a place the team wasn’t expecting it. Song realized that she’d been dwelling too much on that old Scotch-tape method, in a sense trying to get chemicals to replicate what the tape does to pull apart graphite crystals. But the answer was elsewhere, in something more like a chemical push, getting in between layers and nudging them apart. The lab created tiny crystal layer cakes by baking tungsten, sulfur and potassium powder together at 850 degrees Celsius. The process embedded layers of potassium within synthesized tungsten disulfide, like crème between cake layers. When immersed in acid, the potassium, an alkali, bubbled. That pushed apart layers of the material, thereby weakening their bond. It was an effect Song had seen in her other experiments but hadn’t thought of bringing to this research. She saw the opportunity to amplify the bubble effect by applying the mild, shaking waves of sonication. Through further trial and error, the process produced 2D nanolayers that became, so to speak, the quantum leap in a five-year quest.
“I think I keep the team inspired by always reminding them that, if you’re a good scientist, there’s never an experiment that’s a 100% failure,” Schoop said. “Even if your initial idea fails, the really good scientist will see what else is interesting there that shouldn’t be.”
The lab’s atomic-resolution microscope revealed far cleaner results than naysayers predicted. Suspended in water, the nanosheets they made resembled a dark “ink” that when applied to a surface and dried could conduct electricity. Tests showed the printed ink expelled a magnetic field, and its electrical resistance dropped to zero at low temperatures — indicators it was a superconductor.
Song said that when she and Singha put the ink into testing equipment and saw its electronic resistance disappear, they were elated. “We were so excited we almost hugged,” she said. The versatile ink remained robust when exposed to air and potentially could be mass-produced. Applications of technology like this could include electronic devices that bend and stretch, energy-efficient data storage and qubits that will profoundly boost computing power.
For Schoop, it wasn’t so much a “eureka” moment; rather, it was a vision fulfilled. “When Xiaoyu showed me the beautiful superconducting transitions of the films she made from the ink, I wasn’t really shocked, because I was just so sure it would eventually work,” Schoop said. “But I was certainly happy.”
“That’s actually the thing that I learned most from Leslie, the biggest benefit from my graduate studies,” said Song, who now is conducting postdoctoral research at Columbia University. “A lot of good science is unpredictable, and it’s actually a good thing that something doesn’t go as expected. Because our job is to kind of break the boundary of our human recognition, right? Whatever we could predict is within that recognition. Whatever happens beyond what you would predict is actually a breakthrough.”
Being able to recalibrate and embrace the unpredictable is more important than ever with quantum materials, which continue to surprise scientists with properties once considered impossible. Schoop’s team published their findings in 2023 as convention-busting, proof-of-concept research that will contribute to new discoveries, quantum materials and game-changing products.
“Science should never say that something is impossible,” Schoop said. “We can say things are unlikely. But I never like it if somebody tells me I don’t need to do the experiment because they already know it’s not going to work. You need to do the experiment. Then you can tell me if it works or not.”